In a groundbreaking scientific study published just two months after the explosion, Charles Aouad, a Lebanese astrophysicist from Liverpool John Moores University’s Astrophysics Research Institute, specializing in supernovae, and the primary author of the paper, collaborated with a team of physicists and engineers from various affiliations to investigate the event in detail. Their findings reveal that only about 500 tons of ammonium nitrate actually detonated at the port on August 4th, 2020. This is significantly less than the quantities initially reported in the media, shedding new light on one of the most catastrophic events in recent history.
WHAT IS AN EXPLOSION?
When a material burns, it releases energy in a controlled and gradual manner. However, not all burning substances result in an explosive reaction. For instance, wood can burn and generate a significant amount of energy, but even when burned in large amounts, it does not cause an explosion. Similarly, even though butter (yes, the same butter we use in our kitchens) has more energy per unit mass than TNT, it does not explode during digestion because the energy is released slowly. Explosive materials such as ammonium nitrate or TNT release energy instantaneously when burned, because the burning happens supersonically (i.e., detonation). This rapid release of energy causes a shock wave that is incredibly destructive. The shock wave is a concentrated area of the atmosphere where density and pressure abruptly rise (discontinuously), unlike a sound wave where the changes happen continuously. As the shock wave moves at supersonic speeds after the detonation, it deposits energy and creates significant destruction. During a chemical explosion, the hot gases produced from the chemical reaction rapidly expand and exert pressure on the surrounding atmosphere. This creates a rapidly expanding fireball that generates the shock wave. Therefore, the boundary of the shock wave can be traced by following the fireball but only in the initial milliseconds of the explosion when both boundaries overlap. Later, the fireball slows down and stops, while the shock wave continues to expand and cause damage. Physicists can examine the evolution of the fireball to calculate the amount of energy that has been transformed into the blast wave.
HOW WAS THE AMOUNT CALCULATED?
To calculate the energy of the explosion that contributed to the blast wave, the researchers utilized videos captured and shared on social media that depicted the fireball’s size and evolution within the first 170 milliseconds of the explosion. These videos were taken with smartphones from various distances and angles, which the researchers calibrated using the grain silos building as a reference point. They located the video shooting positions on a map of Beirut (see Fig1) and determined the incident angle towards the main façade of the silos building. By de-projecting the silos building based on its known dimensions and applying trigonometric corrections to each footage (see Fig2), the researchers assigned a pixel scale to each frame. This allowed the conversion of pixel measurements to actual physical measurements in meters. The videos were then divided into frames separated by 33 milliseconds, based on the fact that smartphone videos capture 30 frames per second (FPS) (or 60 FPS for some models) (see Figs 3). By measuring the fireball’s size at intervals of 33 milliseconds until about 170 milliseconds, the researchers were able to determine the amount of explosive material that had detonated (see Figs 4&5). The results suggest that only 500 tons of ammonium nitrate detonated, with an energy release in the blast of approximately Joules, equivalent to approximately 200 +/- 80 tons of TNT. (Taking the error margins, this is equivalent to 2-3 percent of Hiroshima Little Boy). This finding is significant because it may suggest that the actual amount of ammonium nitrate that detonated in the warehouse was much less than what was reported in the media. The results of this study were submitted for publication in the scientific journal “Shock Waves,” a prestigious peer-reviewed journal that specializes in the science of shock waves, just two months after the explosion, before the release of the FBI report. Another paper led by professor Yehya Temsah and Dr Ali Jahami, who were co-authors on the first paper and affiliated with Beirut Arab University, estimated the same results using numerical simulations and analyzing the impact damage on the existing grain silos building. Both studies were the first to claim that the actual quantity of ammonium nitrate that had detonated was less than what was initially claimed. These results will be presented at the 34th International Symposium on Shock Waves (ISSW34) in South Korea in July.
For the full scientific papers check here:
https://doi.org/10.1007%2Fs00193-021-01031-9 https://doi.org/10.1016%2Fj.engstruct.2021.112671
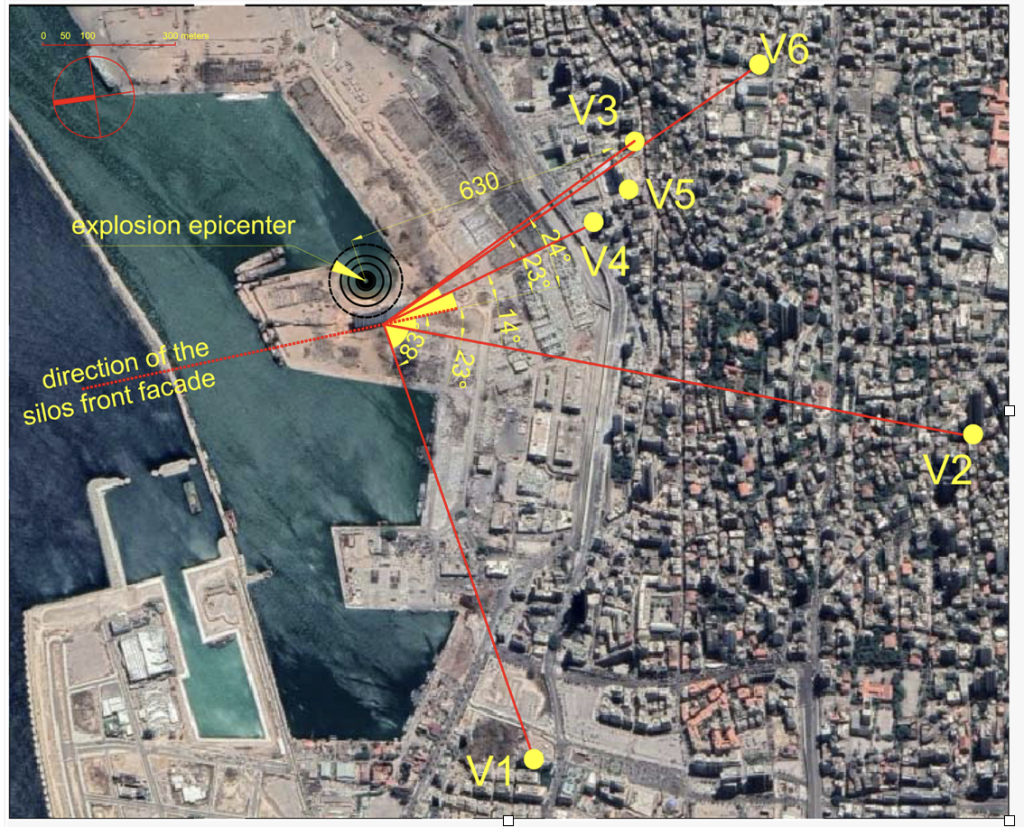
Figure 1 A Google Earth map of Beirut showing the location of the six videos used in the current report. For each video, an incident line of site is determined along the long facade of the grain silos building. The explosion center is marked with black circles
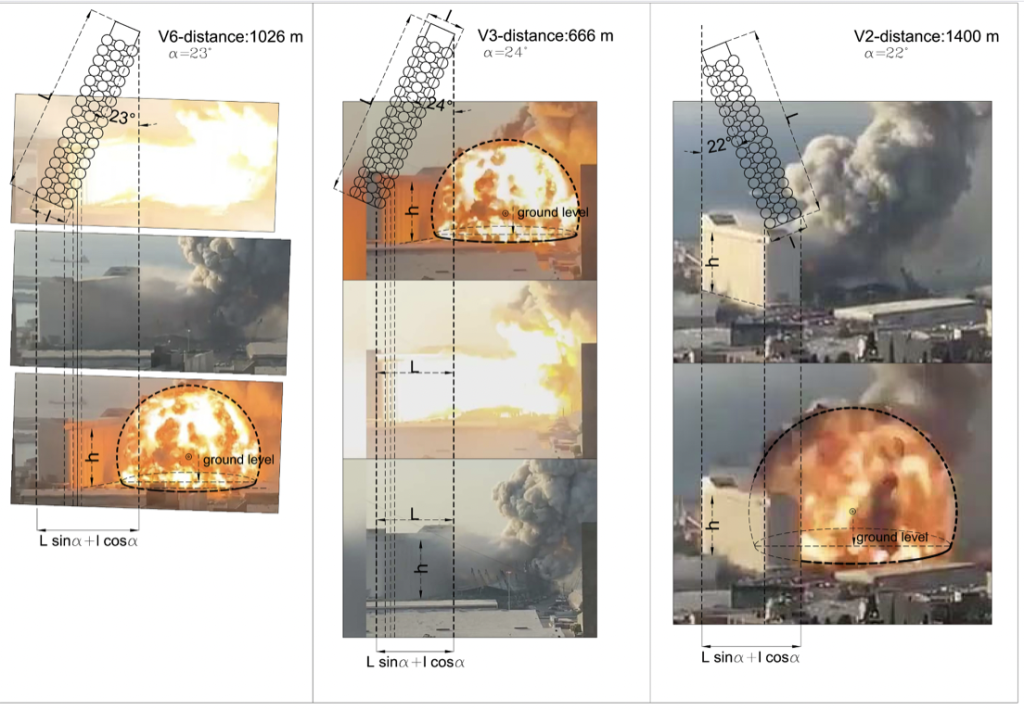
Figure 2: For each video, the incident angle-of-sight toward the silos long facade is shown along with the actual picture of the silos and the projection lines. For complete mathematical derivation check the original article.
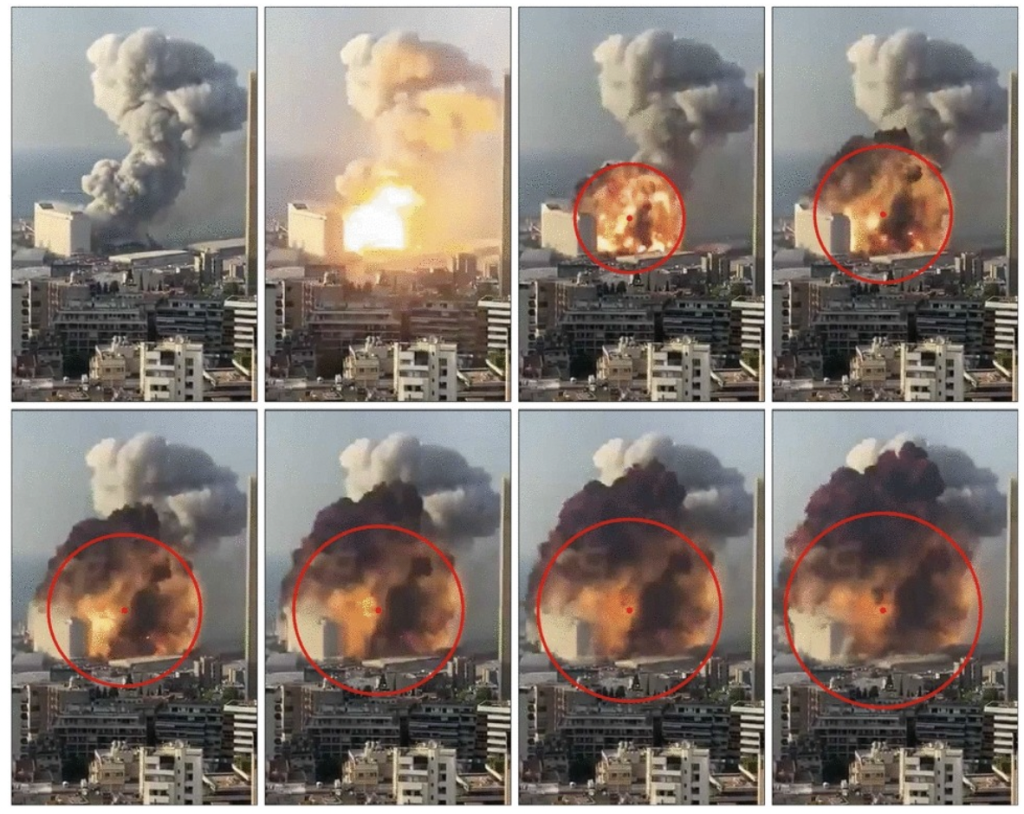
Figure 2 Video V2 taken from 1400 m distance. Eight frames separated by 33.33 ms show the fireball along with the circle fit. The detonation is assumed to have happened anytime between the first and the second frames
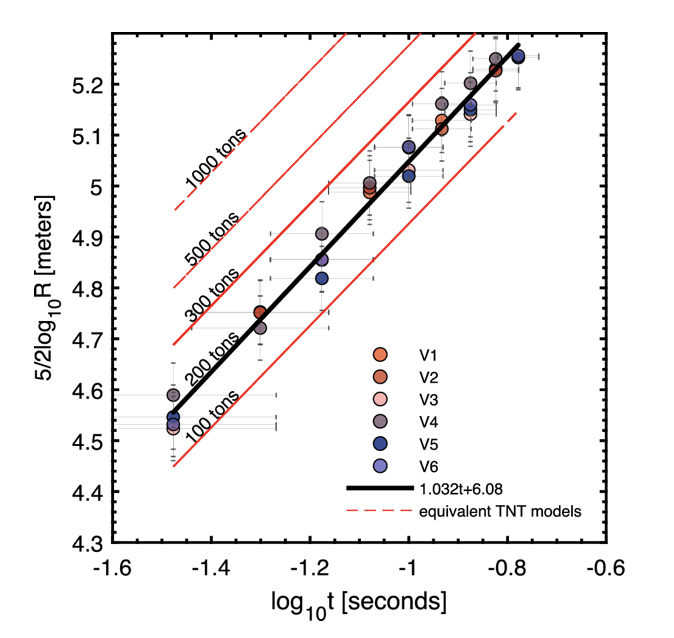
Figure 4 Fireball radius evolution with time; (5/2)logR on the Y-axis and log t on the X-axis. The dots represent the fireball radius taken from different videos for data points taken at a distance less than about 130 m and at times less than about 170 ms when the fireball is assumed to trace the shock wave. The best linear fit has the form Y=aX+b, where a=1.024[0.955–1.092] and b=6.052[5.977–6.127]. The red lines represent the equivalent TNT yields from surface blast explosions, i.e., corrected by a factor of 1.8 to include reflection from the ground. Beirut explosion data is consistent with 200 tons of TNT. This is equivalent to 500 tons of ammonium nitrate.
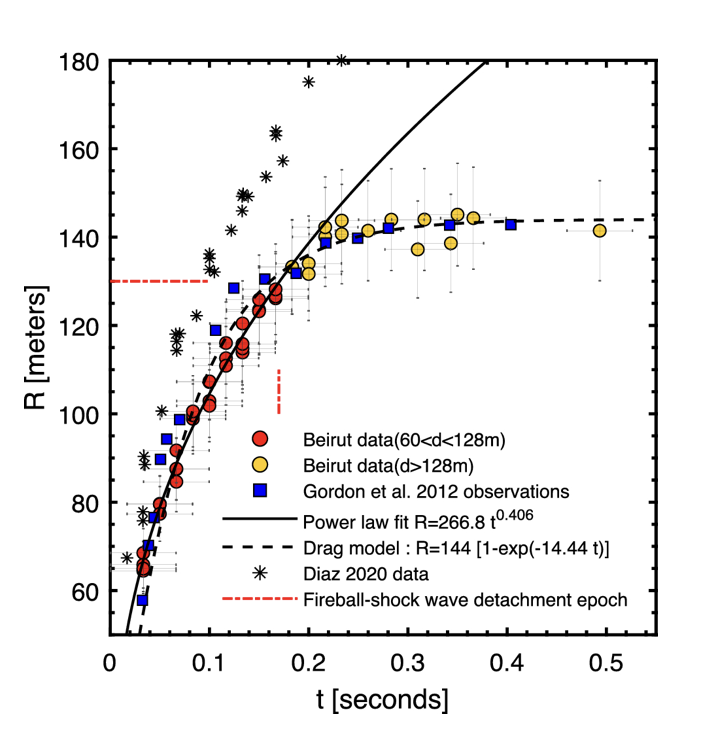
Figure 5 Fireball radius evolution with time. The red dots are the fireball measurements used in evaluating the TNT equivalence of the explosion, taken at distances less than about 130 m and at times less than about 170 ms. A power law with an exponent of 0.406, which is represented with the black line, fits the data within this time interval and is consistent with the Sedov–Taylor model with spherical geometry and instantaneous energy release. Within the above-mentioned time interval, the fireball measurements are assumed to trace the shock wave evolution. The orange dots are the fireball measurements taken at later time moments, i.e., when the fireball and the shock wave have already detached, until the luminous fireball has reached its final radius. The dashed curve represents a drag theoretical model describing the evolution of the fireball until reaching the stopping distance at about 140 m. The straight dashed red lines represent the time moment and the distance where the shock wave is assumed to detach from the fireball. The blue squares represent the fireball evolution in time from field experiments scaled to the TNT equivalence of the Beirut explosion. Small asterisks show the data from other studies.
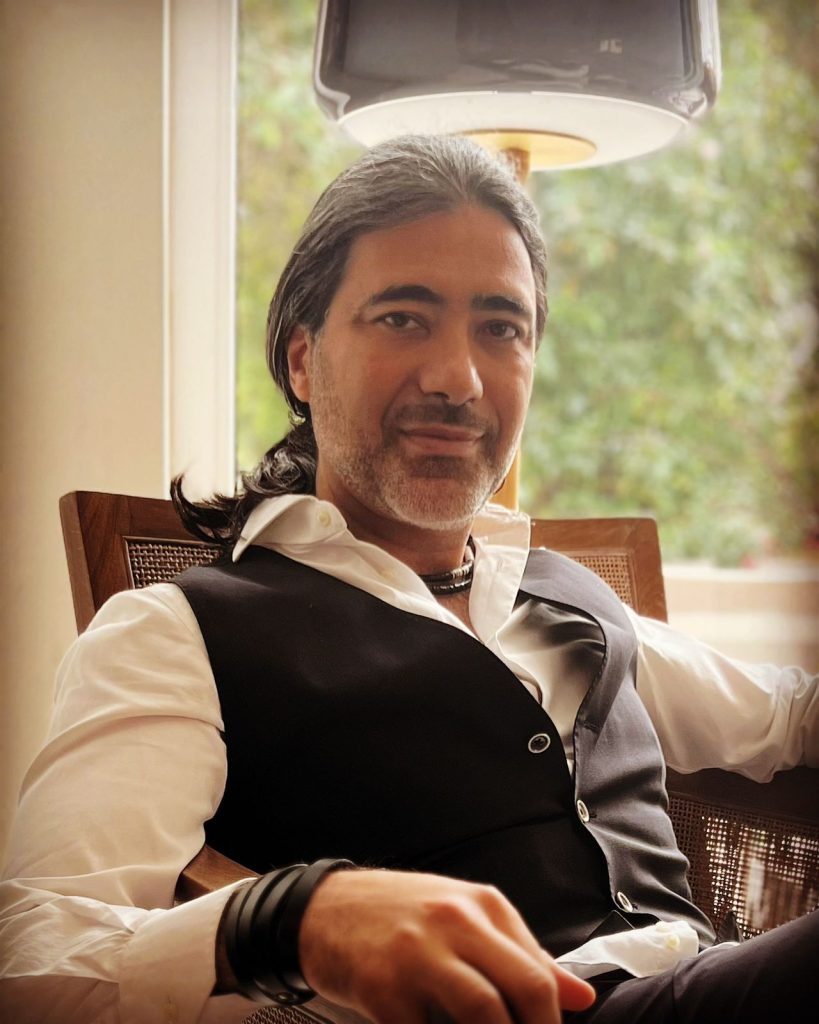
ABOUT THE AUTHOR OF THE ARTICLE
Charles Aouad is a PhD candidate and researcher in astrophysics at the Astrophysics Research Institute (ARI) in Liverpool John Moores University (LJMU), UK. He holds a DES (diplome d’études supérieures) in architecture from ALBA-BALAMAND (1998), an MBA from ESA-ESCP/EAP (2003), and a Masters of Science in astrophysics with distinction from LJMU (2017). His research focuses on theoretical numerical simulations of the spectra of supernovae type Ia, aiming to gain a deeper understanding of their spectral diversities and the viable channels for their explosion-progenitors. His work has the potential to contribute significantly to the field of Supernovae by shedding light on the science of these spectacular thermonuclear stellar explosions.